You’re a mammal. I’m a mammal. Your pet cat or dog is a mammal, as are whales, lemurs, pandas and polar bears. But what exactly is a mammal, and what can our genes tell us about our evolution? Plus, school students take on the whipworm genome, the surprising genetic diversity of Papua New Guinea, and a gene of the month that’s up all night. This is the Naked Genetics podcast for October 2017 with me, Dr Kat Arney, brought to you in association with The Genetics Society, online at genetics.org.uk
In this episode
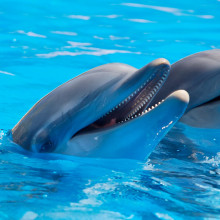
What makes a mammal?
with Liam Drew
From tiny shrews to enormous blue whales, polecats to polar bears, mammals have expanded across the planet to fill a wide range of environmental niches from the frozen Arctic to the fiery desert. But what actually makes a mammal a mammal? That’s a question that bothered neuroscientist-turned science writer Liam Drew when he accidentally took a very painful football to the groin while playing goalkeeper - as only mammals keep their vital reproductive organs dangling about on the outside.
Luckily this didn’t stop him becoming a father, and during that process he became fascinated by the underlying biology - a live baby growing in the womb, fed through a placenta, and then nourished on milk produced by the mammary glands from which mammals get their name. You might think of other traits, such as having fur - but if all it takes is hair and milk, then is a coconut a mammal? To get to the bottom of this mammalian mystery, Kat Arney caught up with Liam at the Wellcome Collection in central London for a chat about his new book on this very subject - I, Mammal.
Liam - You look it up in the dictionary and it says a mammal is a warm-blooded vertebrate with hair and mammary glands, maybe a few other traits if your dictionary is a good one. And so, I thought you know, to define what a mammal is, I’ll write a list of the traits we have hair, a unique type of cerebral cortex, three bones in our middle ears, a unique jaw joint... And I figured that each chapter of the book would describe fairly independently how each of these traits had evolved. And so, it was great fun, looking at how milk had evolved or…
Kat - Boobs and willies, you know.
Liam - Yeah, well I guess I was inspired by reproductive biology, so it did start in the bathing suit area and I’d like to try and move beyond that...
Kat - This is a scientific book obviously!
Liam - Yes, exactly. Well, I did end up trying to arrange the book, having started with the scrotum and saying, “Look! Life begins when sperm are made there and obviously, when eggs are made in the ovaries.” And then I tried to trace the arc of a mammalian life. But to go back to your question, I have this list of traits and this was very 18th century really. You know, the actual classification of mammals can be traced to Linnaeus in the mid-18th century, 1758.
Kat - He did love a bit of classification.
Liam - He did love a bit of classification! And so, it’s in the same book that he coined the term Homo sapiens, that he coined the term mammals. But anyway, next to these 200 primarily European mammals, he had a list of the traits they had and that’s how they did it then. And of course, Darwinian Theory a hundred years later really turned everything on its head. But I think what was important in writing the book, in trying to come up with what a mammal was, was that the chapters weren’t independent at all.
When I was writing about the scrotum, it wasn’t like this made sperm production better. It was like, this is a necessary adaptation to either mammals becoming more warm-blooded or essentially because they started galloping in a certain way which made the abdomen susceptible to these waves of pressure. So we have these two traits of the animal which changed its characteristic.
And then once you went to mammary glands, the sort of main theories on why milk evolved was that before it was a food stuff, it was a useful secretion to either keep eggs moist or to stop from dehydrating, or that the secretion contained components of the innate immune system which killed microorganisms which would have proliferated more on eggs as mammals became warm blooded. So you have this warm-blooded animal laying warmer eggs that needed to be protected either from dehydration or from microorganisms. And so again milk was tied to the warm bloodedness.
There was this fantastic essay written in 1977 on why lactation was important - it was actually published in a month when I was actually being breastfed, which I kind of liked that connection - by Caroline Pond at Oxford University and she listed how as soon as milk could have evolved it changed the landscape again for the possibilities of the animals that possessed it. So suddenly, by the newborns being fed milk, they didn’t have to hunt their own food. And so, they didn’t need such good teeth and so they could grow to a sort of fairly adult size and have an adult jaw and only grow their teeth in an adult jaw.
Mammals have one set of milk teeth and an adult dentition. One of the really defining things of mammals is how sophisticated their teeth are. And one of the things about them is that they interlock perfectly between upper and lower jaws which allows us to chew food very efficiently which helps power this warm blooded metabolism of ours. So again, it was just sort of link upon link. So as soon as I got to the warm-blooded chapter, it was like, “Hey, remember. All these other stuff was spoken about.”
Kat - You can't forget any of it. It seems like being a mammal is absolutely not this like 18th century tick list of, if you’ve got all seven of these, you're definitely a mammal. It’s more like kind of, “do you fit into this weird Venn diagram?” because there must be exceptions. There must be mammals that are weirdly exceptional.
Liam - So, as soon as you say a mammal is an animal with hair, you have a problem with dolphins – they have hair in utero and a few hairs around their mouths when they're first born. But then they have…
Kat - Yeah, like a tiny mustache.
Liam - Yes, apparently useful for finding the mother’s teat. But the dolphin has lost that hair. It’s lost this definition or trait, but it’s still defined by its high energy level lifestyle and all these other traits which allow it to function.
Kat - When we’re talking about Linnaeus, we’re talking about the idea of what is a mammal, Linnaeus and the taxonomists, they love to classify things and say, “Well, it’s got this structure and that form, and so, it must be this kind of animal.” But now, we have genetics. What is genetics telling us about what it is to be a mammal and where we came from?
Liam - When people starting doing taxonomy after Darwin, which was based upon shared history and inter-relatedness, the groups look quite similar with what Linnaeus had come up with because one sort of assumed that a shared physical characteristic meant you are very closely related.
Kat - If you look like a mouse, you're probably some kind of like rodenty mousey thing.
Liam - Yeah, precisely. For a century after Darwin, people were doing taxonomy on morphological basis. And then in the late 1950’s and early ‘60s, this idea of using – it was actually Francis Crick who, in a 1957 lecture said we can start using protein sequences or DNA sequences to trace relatedness. Instead of looking at morphological features, look at protein or DNA sequences of different species and and then further interrelatedness from that.
And it was a wonderful idea. Linus Pauling did this with primate hemoglobin and of course, DNA sequencing was incredibly arduous and hardwork for a long time. Some of the morphologists didn’t really trust this and you can kind of imagine that, right? People who know animals incredibly well and infer everything from all their features, suddenly, guys with test tubes of DNA are like, “Hold on buddy. I've got this covered.” People who’ve never even held an animal could use DNA to infer inter-relatedness.
Kat - It must be a bit like having a paternity test going. “No. That’s definitely my kid.” And it’s like, “DNA says no. I'm afraid that is not a rodent.”
Liam - Exactly. And so, this back and forth went on and on, and in the early ‘90s, some molecular biologists said, “Actually, we don’t think a guinea pig is a rodent” and everyone just sort of dismissed it as very silly. And then suddenly, in 1997, this group in California led by Mark Springer suddenly published this paper entitled, “African Mammals Shake the Mammalian Family Tree.” They basically showed on really robust genetic evidence – they’d really assembled more genetic evidence than anyone had ever previously done – and they suggested that actually, there was a group of African mammals which were more closely related to one another than any other types of mammals.
Kat - So, when people started to discover that DNA was telling us different stories about mammalian history than the fossils, and then the actual physical shapes of mammals, should this change the way that we think about what it means to be a mammal? Is there a genetic definition of what it is to be a mammal?
Liam - The genetics data is now overwhelming in this classification of four groups. One of the really interesting things that it did is that it really tied mammalian evolution to geography. And so, there was the original African group and then the data showed very convincingly that the South American mammals, the sloths, the ant eaters, and armadillos were very closely related forming another group. And there was a third group which could be subdivided in two which evolved on Eurasia. So it actually tied really nicely together the genetic story and the geography of the world.
Kat - I love how in the book you describe mammals as being almost like luxury items in evolutionary history.
Liam - Yeah and I had a moment - that was actually in Darwin’s garden, I think - I was just wandering around there and it’s a beautiful house and it was a weird moment of thinking, ‘there's a lot of plants there’. I was all alone in the woodland behind his house where he used to walk. I'm just thinking, “Look at all these wood and all these plants.”
I mean, it’s absurd – the amount of energy we use. We’re not energy efficient. 95 per cent of our energy consumption goes on just maintaining our temperatures, maintaining our physiology. Together, we are a luxury. You couldn’t have a world made of mammals. We’re reliant on just eating and consuming all the time.
Kat - We are biology’s luxury.
Liam - Yeah, maybe!
Kat - Liam’s book, I, Mammal, is published by Bloomsbury Sigma on the 2nd November, and is available from all good retailers/
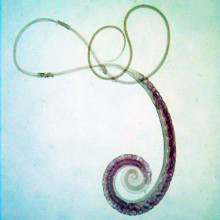
11:10 - Whipping the whipworm genome
Whipping the whipworm genome
with Maria Duque, Wellcome Trust Sanger Institute
The tropical whipworm Trichuris trichiura infects around 500 million people around the world every year, causing diarrhea, malnourishment and developmental problems. At the moment there is no vaccine, and treatments are expensive but pretty ineffective.
To find new ways to tackle whipworm infection, scientists at the Wellcome Trust Sanger Institute in Cambridge have been busy delving into the whipworm’s 15,000 genes, sequencing and annotating the parasite genome. But it’s a huge task they can’t do alone, so they’ve enlisted A level students from more than 60 schools across the UK to help out. Kat Arney spoke to lead researcher Maria Duque to find out more.
Maria - We were very lucky to being told with our collaborator in Denmark, Dr. Peter Nejsum who has infected himself with this parasite. This allowed us to get access to very good material because we can receive the adult worms that actually he had in his intestine and we could sequence that materials and generate and generate a new version of the genome which is much more complete. So, we need to identify where and which had the genes that compose the genome and it is in this endeavour that we look for help from the students, want them to participate to really find out where the genes are.
Kat - So let me get this straight. You have a researcher who has infected himself with this parasite so that you can get the DNA from the parasite and try and assemble all the genes together.
Maria - Yes, but his original objective was actually not to give us the parasite material. He unfortunately has a disease that is called psoriasis. As an alternative treatment, he infected himself with this parasite to decrease the symptoms of this disease.
Kat - Why do you need to get so many children involved in this project?
Maria - Because the genome of the parasite of the human whipworm has around 15,000 genes. So with genomes, you can actually annotate the genes using computer’s algorithms that automatically will tell you which gene is and where the gene is. But unfortunately, when you use computers, the programs cannot really go into the very difficult genes and manually curate the genes. So it’s here where humans are required to really have a very good assembly of the genome to really annotate the genes with precision and it’s here where having a load of students would really help us.
Kat - Something like annotating a genome saying that this is that gene, this is this gene, that seems like something that’s pretty complicated. How are school children able to do this?
Maria - You have a piece of sequence that is just a mix of letters which would be ACGT but then it’s making sense of what this sequence is telling you. So, how can you say in this point of sequence, you actually will have a gene starting and terminating, and how this gene is composed.
So they will be using software called Apollo, they can see the DNA sequence on the upper part. And then they can see the evidence of the genes that means the data that we have obtained after sequencing the RNA. And it’s this sequences from the RNA that gives you clues in the structure of the genes. So, it’s not about which gene is what, but how the gene is composed and how it’s organized.
Kat - So it’s working out kind of what's the important stuff and what's not that important.
Maria - Exactly.
Kat - Why do you need to annotate this genome? What are you going to do with it once you’ve got all the ins and outs, the bits and bobs of the genomes sorted out?
Maria - Having the genome of a parasite or a pathogen is very important because when we do experiments to understand how the parasite infects and how it actually causes disease, this is all due to proteins that are expressed by the parasite and understanding which gene actually is coding for that protein then allows you to do additional experiments. For example, to remove the gene from the genome of the parasite and then study what happens with the parasite when it’s a lack in that gene.
We are now able to move forward into the studies of this disease without having a proper version of the genome so that we can understand in detail how the parasite invades the gut and how it causes disease so that understanding which genes are clues, we can develop new drugs and vaccines to fight the disease.
Kat - It’s wonderful to think that a load of British school children could be behind a new treatment for a tropical disease.
Maria - Yes, that’s great. It’s good to inspire the new scientists and to know that being given this experience actually promotes as they come into science. But they know there is something else behind annotating the genome. There is actually a cause behind that we really want to fight this neglect tropical diseases. And for us, it’s really important to get their help.
Kat - That’s Maria Duque from the Wellcome trust Sanger Institute, and you can find out more information about the whipworm project here.
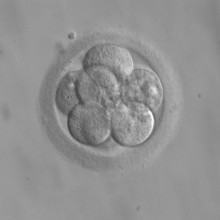
17:08 - UK scientists edit human embryos
UK scientists edit human embryos
Last month scientists at the Francis Crick Institute in London announced that they had successfully used the CRISPR gene editing technique to disable a gene in human embryos - the first time the technique has been used in the UK.
The gene in question makes a protein called Oct-4, which plays a vital role in making sure that stem cells in the early embryo remain as stem cells, and don’t differentiate into more specialised cells too soon.
The team, led by Kathy Niakan, used around 60 donated embryos left over from IVF, which would otherwise have been thrown away, and only grew the modified embryos in the lab for around a week before destroying them. Publishing their findings in the journal Nature, they discovered that modified embryos lacking Oct-4 failed to grow into blastocysts - the football-like stage at which they would normally implant in the womb - proving the gene’s vital role in early development.
More than 80 per cent of human embryos never result in a live birth, so using CRISPR to understand more about the role of particular genes in early development should help to shed light on the misery of miscarriages and infertility, and improve the effectiveness of IVF.
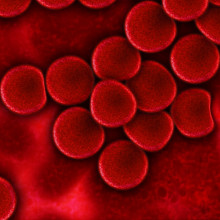
18:27 - Chemical surgery to fix faulty genes
Chemical surgery to fix faulty genes
Scientists in China have revealed details of a new gene modifying technique called ‘chemical surgery’ or base editing, using the method to fix a genetic mutation responsible for the serious blood disorder beta-thalassaemia in human embryos.
Published in the journal Protein and Cell, the procedure uses similar molecules to the gene editing system CRISPR, but only alters one specific ‘letter’ or base in DNA, rather than repairing, removing or replacing longer stretches of sequence.
Many patients with beta-thalassaemia have a single letter mutation in a particular gene, and the Chinese team used this particular change as a model to test the efficiency of their method.
In total, around a fifth of the embryos had a successful edit, fixing the mutation in at least some of the cells. Although the embryos were not allowed to develop longer than a few days, this would in theory be enough to provide some improvement in the disease.
However, the team also found that in some cases they had managed to introduce new mutations into the gene, rather than fixing it - so clearly more work needs to be done on accuracy and efficiency.
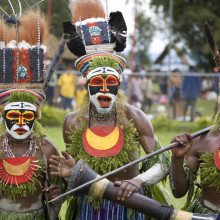
19:52 - Mapping Papua New Guinea’s genes
Mapping Papua New Guinea’s genes
with Anders Bergstrom, Wellcome Trust Sanger Institute
It’s time to take a trip to the Pacific paradise of Papua New Guinea. Humans have lived there for at least 50,000 years, with limited contact from the outside world until relatively recently, and there’s a staggering 850 languages spoken across the country.
Now a team of geneticists have carried out the first large-scale analysis of the inhabitants’ genes, and found surprising levels of genetic diversity. To find out more, kat Arney spoke to study leader Anders Bergstrom - apologies for the slightly dodgy Skype connection, not to Papua New Guinea, but to the Wellcome Trust Sanger Institute just outside Cambridge.
Anders - We’re interested in human evolution and human history and so, we kind of see Australia and New Guinea as, if you will, kind of a second replicate of human evolution. So, you can ask if things unfolded here in a similar way as they did in Europe, Asia, Africa, and so on.
Kat - A kind of microcosm.
Anders - You can say that, yeah. As a bit of extra background, as you’ve mentioned, another reason why we think Papua New Guinea is particularly interesting is that this is one of the places in the world where humans invented agriculture. So, this happened independently a few different times at different places in the world so one is in the Middle East, probably somewhere in China or Southeast Asia. It happened in the Americas somewhere and also in the highlands of Papua New Guinea. All of these things happened in the last 10,000 years but probably independently.
Kat - What did you set out to do and who did you study?
Anders - Together with collaborators from Oxford University and as well as in Papua New Guinea itself, the Papua New Guinea Institute for Medical Research, we had access to an extensive sample collection, DNA samples of people from all across Papua New Guinea.
So, we decided to study these samples genetically so then we could get information from each person on what is the state of their DNA at each of those positions. These data then basically allowed us to ask a whole bunch of questions about genetic relationships, both within Papua New Guinea as well as people in the rest of the world.
Kat - And what did you find when you started to look at all these data together?
Anders - Previously, that’s also been shown that in the coastal areas of Papua New Guinea, there's been some gene flow from Southeast Asia in the last few thousand years. So, in this period they were agricultural people in southeast Asia who sailed down to New Guinea and they mixed with some of the local people in the coastal areas. However, there's a large mountain range that runs through the centre of New Guinea Island. It hasn’t really been known before if this southeast Asian gene flow reached also the people who live in these highlands, in the mountains. And so, what we found was that actually, it didn’t. So the people in the highlands have been completely independent genetically ever since people first reached this part of the world 50,000 years ago, until the present day.
Kat - I love the scientific euphemism ‘gene flow’ when really, you mean people coming, falling in love, having families, going down the generations.
Anders - Yeah, exactly.
Kat - Were there any other interesting findings apart from the Southeast Asian people not wanting to go climbing?
Anders - The most interesting findings actually relate more to the internal history of Papua New Guinea. Another big motivation going into this project was that we know that Papua New Guinea is extremely diverse linguistically. So, this country has approximately 850 languages. This is approximately 10 per cent of all the languages in the world.
Kat - Wow!
Anders: As well as cultural diversity between different groups of people. So, we wanted to know if this great linguistic and cultural diversity was also reflected in the genetic structure of people so that different groups of people who spoke different languages were also genetically different from each other. And so, using this data, we found that this is indeed the case. Different groups within Papua New Guinea are indeed surprisingly genetically different from each other.
So despite this being a relatively small geographical area, the genetic differences between different groups are generally larger than any differences you find for example within all of Europe. So for example, if you compare Spanish people and Finnish people - kind of the two corners of Europe in some sense - many groups within Papua New Guinea are much more different than that.
Kat - Are there lessons from your findings that we can take to other populations? I’m thinking maybe isolated populations like Iceland or other areas of the world.
Anders - Part of the reason why these groups are so different from each other is that many of the groups are relatively small and if you have a small population size then the rate at which you accumulate differences relative to another population is higher. You can see similar effects in other parts of the world. It’s a kind of bottleneck. Iceland is one example but there are many others where small populations accumulate differences faster.
So there's an interesting question that kind of goes back not just in genetics but also spanning archeology, linguistics, and so on, about what happens to human populations once you invent agriculture. And also, how does agriculture spread? Does it spread because the people who invent agriculture expand and replace everyone else? Or does it spread just culturally that you see your neighbors growing plants and then you start doing it too? With Papua New Guinea, we can then kind of ask this question independently, what happened here after agriculture?
Once humans moved from a hunter-gatherer lifestyle where you live in small groups and you move around across the landscape, and instead you settle down, expand and you start to get little villages and towns, and maybe this would over time lead to a more homogenous genetic structure. But actually, Papua New Guinea then shows that this is not necessarily the case because here, we had agriculture too. People did settle down into villages, but they still retain relatively little movements between groups and the groups remain genetically quite distinct from each other.
The real question then is, if both Europe and New Guinea had agriculture, why did Europe end up being very genetically homogenous whereas New Guinea ended up being very diverse? So, we can only speculate about this at this point with the data that we have but what we do suggest is that what made Europe different was that it had a Bronze Age and a subsequent Iron Age, and following cultural processes. And you had similar processes in other parts of the world as well and in East Asia and in Sub-Saharan Africa.
Perhaps it was this later technologically driven expansion that in some sense, changed the culture of these regions. People started traveling more, trading more, and this in the long term then led to more genetic mixing and a more genetically homogenous region. Whereas in Papua New Guinea, there was no Bronze Age, no Iron Age. And so, it might have better kind of reflect the levels of genetic diversity as well as linguistic diversity that many human societies would have had before this Bronze Age or Iron Age expansions.
An implication perhaps or a speculation rather coming out of this work where we see this very strong genetic structure in Papua New Guinea as well as very high linguistic diversity is that probably, many other places in the world would have also been more linguistically diverse in the past. So, if we again return to Europe as a comparison, the Bronze Age in Europe was associated not just with a great genetic turnover and mixture and replacement, but probably also, a linguistic turnover.
So, there's still some amount of debate about this among linguists and archeologists, but the prevailing idea seems to be that with the Bronze Age in Europe which occurred maybe 5,000 years ago, the vast majority of languages within Europe were actually replaced by the languages we speak today in Europe which are the so-called Indo-European languages. There are only a few remaining languages in Europe which are not part of this family. So most likely, before that time, Europe also would have had a much larger number of languages perhaps similar to Papua New Guinea and that they have been then lost and replaced with a single family which is what we speak today.
Kat - Anders Bergstrom from the Wellcome Trust Sanger Institute.
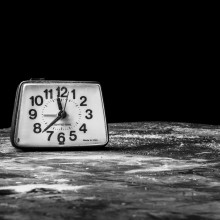
Gene of the month - Insomniac
As you might have guessed from the name, Insomniac is a gene involved in sleep, and was first discovered in 2011 when researchers screened more than 20,000 fruit flies which normally had regular sleep patterns to find mutants who had problems flitting off to the Land of Nod.
Lazy normal fruit flies sleep for an average of about 15 hours out of every 24, but flies with an Insomniac mutation only manage about 5 hours of shut-eye per day. The gene is usually active in the fly’s nerve cells, and seems to be involved in breaking down certain proteins.
Humans spend around a third of our entire lives asleep, and sleep deprivation and chronic insomnia can have severe impacts on health and wellbeing. However, the exact biological function of sleep is still not fully understood, and although Jeffrey Hall, Michael Rosbash and Michael Young have just won this year’s Nobel prize in physiology or medicine for their work on circadian rhythms - the internal body clock - we still don’t fully understand the genes and molecules that control sleep.
Humans and other mammals also have versions of Insomniac, which are found at the connections between nerve cells, known as synapses. Earlier this year, researchers in New York discovered that two different mammalian versions of Insomniac can compensate for the missing insect version in mutant fruit flies, suggesting they probably help to send us drifting off in the same way the original Insomniac gene works in flies.
Sleep problems are common in neurological conditions including Alzheimer’s and autism spectrum disorders, so unpicking the molecular pathways around Insomniac might reveal more about the connections between sleep, brain function and disease. Something to mull over when you’re wide awake in the wee small hours, anyway.
Comments
Add a comment