In this NewsFlash, we meet the deep-sea microbes that feed on the hydrogen from hydrothermal vents, and discover a cheap new catalyst that can generate hydrogen ten times faster than methods found in nature. Plus, how reprogrammed immune system cells can kill cancer, and nature's stock exchange - how plants and fungi develop a free-market economy for shared resources.
In this episode
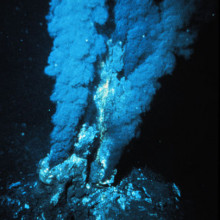
00:19 - Hydrogen fuel cells found in deep-sea mussels
Hydrogen fuel cells found in deep-sea mussels
Mussels flourishing in the dark ocean depths at hydrothermal vents harness energy from hydrogen, thanks to symbiotic bacteria acting as 'fuel cells' inside their gills. This discovery unveils a third energy source, alongside sulphur compounds and methane, that power these sunlight-starved ecosystems in the deep-sea.
A research team led by Jillian Peterson and Nicole Dubilier from the Max Plank Institute for Marine Microbiology in Germany, explored a hydrothermal vent field called Logatchev at the Mid-Atlantic Ridge 3 km beneath the waves.
Superheated water gushing from these vents has the highest hydrogen levels ever measured in hydrothermal systems. Peterson and the team wondered if any organisms were tapping into this potentially rich source of energy - hydrogen is a great electron donor and the energy released by hydrogen oxidation is much higher than for methane or sulphur.
To find out, they sent down remotely-operated submersibles that brought up living samples of mussels, called Bathymodiolus, that cluster around these vents. Back on deck, researchers discovered that sulpur-oxidising bacteria living inside the mussels' gills can also be powered by hydrogen. They have a gene, hupL, that codes for a key enzyme involved in harnessing energy from hydrogen, which essentially breaks down hydrogen molecules into two hydrogen ions plus two electrons.
The team also showed that Bathymodiolus mussels mop up large amounts of hydrogen both in the lab and using in-situ equipment back down at the hydrothermal vent. They found that hydrogen levels were much higher in water spewing straight out of the vent compared to water after it had passed through a dense bed containing up to 500,000 mussels.
Until now, only free-living bacteria have been shown to use hydrogen as an energy source.
Other animals - including the giant tubeworm, Riftia, and the shrimp Rimicaris exoculata - live around hydrothermal vents where hydrogen is less abundant than at Logatchev but it turns out they also have hydrogen-harnessing capabilities. They too host symbiotic bacteria with the hupL gene, suggesting that an ability to harness hydrogen is widespread across vent communities.
The paper appears in this weeks edition of the journal Nature.
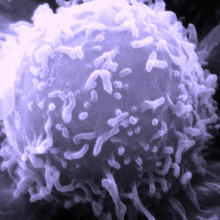
05:07 - Reprogramming T cells to kill cancers
Reprogramming T cells to kill cancers
For the first time, scientists have used genetic engineering to reprogramme immune cells to successfully eliminate tumour cells in one form of human blood cancer.
Writing in Science Translational Medicine, University of Pennsylvania scientist Michael Kalos and his colleagues collected T lymphocytes, a form of white blood cell, from three patients suffering from the disease B cell lymphocytic leukaemia (B-CLL).
In cell culture, a modified virus similar to HIV was used to add to the T cells genetic sequences encoding a signal called 4-1BB, which encourages active T cells to proliferate, as well as an antibody-like structure that could selectively recognise a chemical marker called CD19 that is expressed by the malignant B cells.
Between 10 million and 1 billion of the reprogrammed T cells were reinfused into each of the patients, in whom they persisted at high levels for at least 6 months, relentlessly hunting down and destroying the leukaemic cells in both the blood, bone marrow and lymph nodes.
Follow up tests showed that each infused T cell destroyed at least 1000 leukaemia cells, leading to the destruction of an average of over 1kg of cancerous tissue in each of the patients, two of whom went into complete remission following the therapy.
Previous attempts to use this sort of adoptive immunotherapy, as this approach is known, have been frustrated by the poor long-term persistence of the modified T cells in circulation. This allows the underlying disease to "escape" again, triggering a relapse.
But the researchers believe that the key to their success in this case was the use of the additional regulatory 4-1BB component, also known as CD137. By including this, they think, the modified T cells are stimulated to multiply as they fight the disease, helping to prop-up their numbers.
It's not all plain sailing though. Whilst targeting CD19 ensures that the modified T lymphocytes attack only B cells, this means that healthy, non-cancerous B cells are hit too. And as these cells play an important role in immune defence, including producing antibodies and providing a stored "memory" of infections fought previously, their loss can leave patients vulnerable to infection. This could be avoided in future by developing ways to target the T cell attack exclusively to the malignant cells, but this is still some way off.
It's also important to emphasise that the present trial involved just 3 patients who have been followed up for just under a year so far. The study results therefore need to be interpreted cautiously, although with optimism.
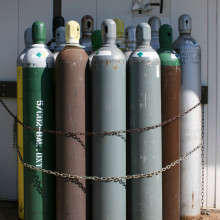
07:55 - Nature Inspires High Speed Hydrogen Production
Nature Inspires High Speed Hydrogen Production
Dr Morris Bullock, Pacific Northwest National Laboratory
Chris - We're joined by Dr. Morris Bullock, one of the scientists behind the work. Hello, Morris.
Morris - Hello.
Chris - Thank you for joining us on the Naked Scientists. Kick off if you would, and tell us, what was the actual goal of the work when you started? Why were you doing this?
Morris - The goal of our research is to develop ways to convert between electrical energy and chemical energy. And the reason is that we all hope that in our future we'll be using a lot more renewable sources, such as solar power and wind power. To make that as effective as possible we need to have ways to store the energy. Of course there are going to be times that we want to have energy usage or electricity available when the sun's not shining, or when the wind is not blowing, and so it's important to have a greater use of these renewable sources, and that we find a way to convert electrical energy to chemical energy.
Chris - So in other words, you put the electricity into hydrogen, and you want to be able to do that efficiently, and then get the energy back out of the hydrogen again later when the sun's not shining.
Morris - That's exactly correct. We're taking electricity. We're doing an electrochemical experiment. We convert the electricity; we take two electrons and two protons and we convert them into hydrogen. That way we store the electrical energy in the form of a chemical fuel that is, in our case, in hydrogen. Then we have that hydrogen. It would be available at the time that you need electricity, and then we would run the reaction in exactly the opposite way. That's what happens in a fuel cell, you run hydrogen into the fuel cell to get electricity back out. And so, the catalyst that we just recently developed converts electricity into hydrogen much more quickly than the previous catalysts.
Chris - What exists in nature to do this and what's wrong with the natural form? Why can't we just use that?
Morris - Well, the ones that nature has are amazingly efficient. They're terrific catalysts, but they exist in hydrogenase, that's found in microbes, and that kind of thing in nature. The problem is they exist in a very specific biological environment and are not often that stable outside of their natural environment.
Chris - So what is it that you've been able to do and how have you used what those bacteria, those microbes with hydrogenases, do to inform building this new catalyst?
Morris - What we've done is to look at what the hydrogenase enzymes look like. Then what we tried to do is to emulate just the important functional features. In particular, we know that the hydrogenase enzymes are based on metals like iron or nickel, and so these are cheap metals as opposed to a precious metal like platinum. The second key feature of what we've seen is that one of the key chemical features of the hydrogenase enzyme is what we call a pendant amine. That's just the nitrogen containing group that has a basic site and that helps to move the protons. What we've done is to incorporate that amine functionality, that basic site to move protons into the structure, and we found that being able to move protons more efficiently makes a huge difference in having a fast catalyst.
Chris - So rather than having to rely on an enzyme, which is protein based and therefore more difficult to work with, you've been able to make a solid state. Basically, a crystal architecture, with various chemicals in just the right places to do this chemical reaction. But how do you build that catalyst? How do you get those chemical groups, including the nickel and the iron you mentioned, in just the right place? So that the reaction happens as well as it does, or better, with the enzyme, but without having to use an enzyme?
Morris - These were just made up in the laboratory, so these are completely synthetic molecules. We assemble a ring containing two phosphorus atoms to bind to the metal, and then between the two phosphorus atoms is a nitrogen that has the amine. And so then we attach that to a nickel. In this case it's just a nickel catalyst, not any iron involved in this so it's just one nickel molecule that has two - what we call ligands - that is a chemical attachment to the metal, and has the amine attached. We synthesise that in the lab and then we do the experiments where we look at the conversion of electricity to hydrogen.
Chris - And how good is it compared with what microbes can do?
Morris - The fastest reported rate for natural hydrogenase enzymes is a turnover - that's we call the turnover frequency - of 9,000 per second. The one that we have recently made turns over at 100,000 times per second, so it's approximately 10 times faster than the natural hydrogenase enzyme.
Chris - And is it economically and scientifically viable to actually make this stuff on the kind of scale that we would need if we were to try and do this industrially?
Morris - I think this one would not be economically viable to use in its present form. We're studying fundamental science and we think that the discoveries that we've made will be helpful in showing us how to take the next step of making one that would be even better, but even though this is a very fast rate, the efficiency is not good enough at this point to really make it so viable for implementation in an industrial type of setting at this point.
Chris - So a little bit more work to do, but still, congratulations - a wonderful study. Morris Bullock there. He's from the Pacific Northwest National Laboratory in Washington State and you can find that paper published this week in the journal Science.
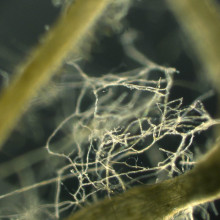
14:05 - Plants and fungi pioneers of free market economy
Plants and fungi pioneers of free market economy
It's not just humans that create market economies, exchanging one set of goods for another - plants and fungi also barter nutrients with deals kept in check by market forces, new research has revealed.
Writing in Science, Vrije University researcher Toby Kiers and his colleagues show how the roots of plants feed sugars to soil-dwelling fungi in return for phosphorus and other nutrients they can't make themselves. This means that plants can effectively make use of the extensive, branching subsoil network of fungal hyphae almost as an extension of their own root systems.
But it's not a simple case of give and take: plant roots can discriminate between more or less generous partners and pay higher sugary prices to those fungi that are more generous with their nutrients, and vice-versa. Consequently, cheapskate fungi are priced out of the market by their more generous counterparts.
The team made the discovery by using radioactively-labelled carbon and phosphorus to follow the exchange of materials between a small clover-like plant called Medicago truncatula and three closely-related species of fungi, Glomus interadices, Glomus aggregatum and Glomus custos.
In an initial series of experiments, the plant roots were grown in isolation on a Petri dish with each of the different fungi in turn. Glomus interadices, the team found, consistently provided the plant with more phosphorus and was consistently rewarded with bigger rations of sugar. Glomus aggregatum was less forthcoming with its phosphorus and consequently received low sugar payments, whilst Glomus custos was downright selfish and supplied the phosphorus in a form that the plant couldn't use and so received little reward in return.
But when the three species were grown together with the same set of roots, would the plant be able to tell who was short-changing it and so apply a penalty to the offender? Incredibly, the answer is yes. When all three fungi were co-cultured alongside the same set of roots, the less generous fungal species were consistently less well rewarded than their more generous counterparts, which effectively priced them out of the market, becoming the dominant nutrient supplier within a short time.
This shows that these sorts of mycorrhizal relationships between plants and fungi, which are thought to have existed for more than 470 million years and may even have enabled plants to first colonise the land during the Devonian era, represent a genuine subsoil free-market economy that Barrack Obama would be proud of!
Comments
Add a comment