Fusion power by 2040?
Today, we're asking whether UK science minister George Freeman's ambition of an operational fusion energy plant by 2040 is realistic. Scientists have their say as to whether or not this possible and, if not, when we can expect to see fusion on grid...
In this episode
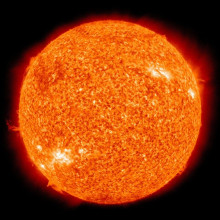
02:27 - Nuclear fusion 101
Nuclear fusion 101
Brian Appelbe, Imperial College London
To kick us off with some of the background to nuclear fusion, we’ve got Imperial College London physicist Brian Appelbe...
Brian - Yes, nuclear fusion at its most basic level is just a nuclear reaction in which you take two nuclei and these are light nuclei that come from near the start of the periodic table and you force them to join together. You fuse them. And when you do that, Einstein's famous equation, e = mc^2, possibly the most famous equation in physics, comes into play. A small amount of the mass of those nuclei that you're fusing together is converted into kinetic energy of the particles. So really you're not creating energy, you're just moving energy from being part of the rest mass of the particles to being a kinetic energy which you can then recover and use to create electricity. But yes, this is different to our current power plants which are all based on fission. Fission is whereby, again, it's a nuclear reaction, but it's when you take the heavy elements from the end of the periodic table, and you essentially cause them to break apart, you bombard them with neutrons, they break apart. And again, Einstein's equation means that that releases energy. So fusion is when we are joining the particles together. The real challenge though is, when we bring the particles close together, they start to repel each other electrically. And what we need to do is we need to make this thing that we call a hot plasma whereby the fusion reactions can actually happen.
Chris -Yes, we know the physics works because we look up in the sky and there is a sun which has been there for billions of years and is responsible for all life on earth, or at least sustaining all life on earth. And therefore we know the physics works. But we basically have to try and recreate that here on earth.
Brian - I think that's very true. And sometimes us fusion physicists question, are we engineers or physicists? So this is the process by which the sun gets all its energy. So we know it works. What we are trying to do is do it in a controlled manner, at the moment in the laboratory. Can we essentially get these reactions to happen on a small enough scale such that we can actually recover the energy and convert it into electricity. So that's really challenging because when you make this plasma hot, it immediately wants to blow itself apart. The sun works because it is so massive that the gravitational force from all the massive material in the sun holds it together. But when we make our plasmas in the laboratory hot, they tend to just blow apart. So we must find ways of confining them and keeping them in place.
Chris - Why is this regarded as such an energy panacea? Why is this better than the fission process that we know we can already do, do well and produce lots of energy that way?
Brian - It's a nuclear form of energy. So that means it's not going to really emit many greenhouse gases as compared to fossil fuels. Then comparing it with fission, essentially you get more energy per unit mass from fusion than from fission energy. So it's a more efficient source of energy. And then also because you're taking nuclei that are near the start of the periodic table, they are less radioactive than the fission materials that you use. So the nuclear waste from fission, we have to store that for thousands of years. And I think the final point contrasting it with fission is we feel that it is a safer form of energy in the sense that there is zero chance by design and the way the fusion reactions work of any sort of nuclear meltdown in the types of power plants that we would have.
Chris - Interesting. When you mentioned that we're fusing smaller elements to make bigger ones, and scientists often talk about using particular forms of hydrogen, heavy hydrogen like deuterium and tritium to do this. Why are we fixated on that recipe? Can we not just take any old element from the periodic table and fuse it with another one and make a bigger one and get the same process?
Brian - Well, we could up to iron. So iron is element number 27 in the periodic table. All the elements up to iron, if we fuse them together, we will release energy. But there are two specific reasons why we take D and T, these isotopes of hydrogen. And that is because first of all, they've a very large cross section and what we mean by cross-section is the probability when you bring them close together that they will actually fuse.
Chris - This is deuterium and tritium?
Brian - The deuterium and tritium, yeah. They're isotopes of hydrogen. So that's one reason. And then the second reason is how much energy is released when you actually have the fusion reaction? And so the deuterium tritium D, T pair is the pair that releases the most energy per reaction happening.
Chris - So we get the best bang for our buck?
Brian - We get the best bang for our buck.
Chris - When we were thinking about harnessing energy that was in say, oil or coal hundreds of years ago, we built an engine that would liberate the fuel in the right way. What sort of engine should we be building for liberating the energy that's in fusion fuels like you've just been describing? What should that engine look like?
Brian - So I think the engine is probably really dictated by these constraints that we have that we need to make something very hot and then keep it in place for sufficiently long time for these reactions to happen. And so there's at the moment two different designs or concepts for these engines. There's one form called magnetic confinement - and I believe we'll have someone speaking a little bit more about that later - in which you have this hot deuterium, tritium plasma and then you wrap it in magnetic fields. And these magnetic fields keep it in place while the reactions are happening and it produces the energy. And the other form of engine is something that we call inertial confinement fusion, where we just compress the fuel as rapidly as possible. And doing that compression makes it very, very hot. And then we aim to get as much of the fuel to burn up in as short timescale as possible.
Chris - And what do you think are the big hurdles that need now to be surmounted to reach George Freeman's 2040 goal?
Brian - At the moment when these reactions happen, we produce a burst of neutrons, high energy neutrons, and going from creating a burst of neutrons to having electricity is a non-trivial challenge. It involves a lot of material science that we have yet to do.
Chris - Brian, thank you very much. That's Brian Applebee. He's staying with us across the programme. We'll hear more from him in a minute.
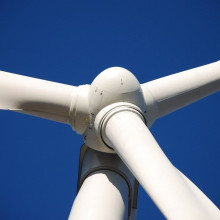
08:15 - Do we really need fusion?
Do we really need fusion?
Ian Lowe, Griffith University
It does seem as though optimism for fusion has surged recently. But nuclear physicist and emeritus professor at Griffith University in Australia Ian Lowe is much more sceptical of the recent bullish predictions around the future of fusion power…
Ian - It does not seem plausible at all. I remember the standard joke among physicists is that commercial fusion power is 50 years away and probably always will be. In the 1960s, fusion was just a laboratory activity and the achievement of briefly getting out more energy than was put in is a significant achievement in terms of the basic physics. But it's a long way away from being a plausible commercial activity. I haven't yet seen a credible conceptual diagram for how you structure a reactor so that you maintain the plasma at the temperature of millions of degrees, which is needed for fusion to go on at the same time as extracting usable quantities of energy. I don't think it will be commercially realistic in my lifetime.
James - Interesting you say that. So what's caused this excitement, this generated buzz around the topic, do you think?
Ian - Well, what's caused the excitement was that one experiment in which, for a few milliseconds, more energy came out of the reaction than was put in,
James - That was at the National Ignition Facility in America. Just to clarify, wasn't it?
Ian - Correct. And there's been a lot of hype about that, but it's important to remember that while the amount of energy that came out was slightly more than the energy that went in, putting that energy in required the amount of energy that would power a small city. So what we're talking about is an experiment in which the amount of energy that would power a small city briefly produced enough energy to run half a dozen households. Now that's interesting in terms of basic physics, but it's a long way away from being the commercial reality of providing the low carbon electricity we need if civilization is to continue without causing runaway global warming.
James - Well, I suppose then we've got an energy gap to fill, haven't we? We've seen a lot of progress in other renewable energy sources in recent years, solar and wind. Is the sort of buck going to be passed to solar and wind?
Ian - If we're talking about zero emissions by 2050. The easy part of that is getting zero emissions from the electricity supply. It's much harder to decarbonize transport and agriculture and buildings and manufacturing. So we really need to have zero carbon electricity by 2040. And if the earliest that the most optimistic view of when you could have one commercial reactor running is 2040, that just doesn't make any impression at all on the problem.
James - I wonder if you could speak a bit to the deployment difficulties though with solar and wind and that that's been the sort of root cause, hasn't it, of why people have looked at ventures like fusion as somewhere we need to find energy if we're going to, as you say, decarbonize by our ambitious global targets.
Ian - Yes, I suppose there's two points. One is that in the last decade there's been dramatic reductions in the price of electricity from solar farms and large wind turbines. In 2010, the average price of nuclear efficient electricity was about 12 cents a kilowatt hour. Wind was about 14, solar was about 35. Last year, world average prices were 3.70 cents for solar, 4.1 for wind, eight for gas, 11 for coal, 16 for nuclear. So solar and wind are now far cheaper and they're so much cheaper that in the northern hemisphere, large coal and nuclear power stations that have long ago amortized their capital cost are being closed down. The critical point of using solar and wind is that you needed storage because the sun doesn't always shine and the wind doesn't always blow. There's been great improvements in battery technology and pump hydro storage. The other point I'd like to make though is that we shouldn't assume that the demand for electricity is fixed and given. By far the most cost effective way of reducing the amount of carbon dioxide we put in the atmosphere is improving the efficiency of turning energy into the services that we need. The American energy analyst Amory Lovins said 50 years ago, people don't want energy. They want hot showers and cold beer. And we really should be putting a lot more effort into improving the efficiency of the appliances that turn energy into the services that we need, like heating and lighting and electronics, rather than assuming that we need to keep scaling up the amount of electricity that we pour into inefficient devices.
Chris - Ian Lowe. Slightly surprised he didn't mention the fact that, here in Britain, we of course have cold showers in warm beer, but there we are. Imperial College's Brian Applebee is still with us. He made some pretty strong points there. Do you agree?
Brian - Well, I think I would agree with his, you know, assessment that there are a lot of technological challenges and we have to be open to that. And I think it would be very reckless of anyone to assume that fusion energy is going to help us with getting to net zero. You know, there is no way that we can rely on it.
Chris - As in, because it's not gonna happen soon enough.
Brian - Well, it may or may not, but I don't think we can assume it will happen by 2040 simply because there are so many technological challenges that we have not yet tackled. I think we can be confident when we have the time and the resources to tackle them that we will be able to overcome them, but that might take longer than we expect. And so I think he's right on that point. But I think just stepping back from the purely commercial point, you know, it's a challenge worth undertaking because it is such a fundamentally different form of energy. So if we can get it to work, it is game changing.
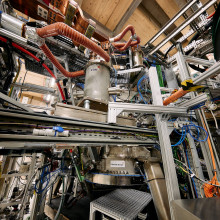
14:53 - Let's talk tokamaks
Let's talk tokamaks
Hannah Willett, Tokamak Energy
Let’s shine the spotlight on one of the ventures endeavouring to solve the practicalities of actually making it happen. James heard from Hannah Willett at Tokamak Energy in Oxfordshire. They’re using the same technology as the STEP 2040 prototype plant will…
Hannah - Our approach is called magnetic confinement fusion, and essentially imagine trying to hold a jelly in a net except our jelly is our plasma fusion fuel - the hydrogen - and the net is made of magnetic field lines. So we have a machine called a tokamak which is a vacuum chamber in a kind of a cored apple shape. Inside this vacuum chamber, we suck as much air as possible out of it, put our fusion fuels inside it, heat it up, strike up a plasma as it's called and we use our magnetic net. So we have lots of field coils around the outsides of the chamber to hold the plasma inside, keep it away from the walls, make it stay nice and hot for the fusion reactions to happen.
James - A really nice minute long introduction to how it works. I wonder if we can unpack that slightly. Let's start with the inputs of the process. You need those hydrogen isotopes to smash into each other. Now where do these come from?
Hannah - The hydrogen isotope that is most common is hydrogen (1H), which is one proton and one electron. What we'll need to use for fusion on earth is deuterium and tritium, which are slightly heavier isotopes. So for deuterium, you add one neutron and for tritium, you add a second neutron. Now, deuterium is still naturally occurring. It's about one in every 6,700 hydrogen atoms, which doesn't sound like much, but when you consider how much hydrogen there is on earth, mostly in the form of water, H2O, in every cubic metre of seawater there's 33 grams of deuterium atoms. Tritium is a little bit more awkward, so it's slightly radioactive. It doesn't hang around forever. That will need to be produced, which is one of the, the big technological challenges of fusion. But it can be produced from lithium. So designing your power plant to be able to manufacture tritium as well to feed back in, that is part of the development of technology that needs to happen.
James - But one of the other major challenges is that enormous, extreme amount of heat you need to generate to get the deuterium and the tritium into a plasma and to keep it in that state. It needs to be millions of degrees, even hotter than the sun. How are you doing that? Where are you doing that? That's where the tokamak comes in, I assume.
Hannah - Exactly, yes. So the reaction rate does depend on the temperature. So the reason we have to reach these temperatures several times hotter than the centre of the sun, these millions of degrees, is because the reaction is most efficient at those temperatures. Fusing two nuclei together, they're both positively charged. So there's an electrostatic repulsion between them. They have to have enough energy to be able to be pushed close enough. Together we have neutral beam heating, which means that we create high energy beams of neutral hydrogen or deuterium, give them loads of energy, fire them into the plasma, and then once they're inside the plasma they have to be neutral to be able to get inside that magnetic net. But once they're in, they interact with the plasma particles that are already there and transfer that energy and actually allow that fusion reaction to happen.
Hannah - So the temperature is a big part of it. The other thing is density. Basically the denser your plasma is the more particles you have flying around in there and the more likely they are to interact. So there's kind of a careful balance between temperature, your density, and then the third element of N for density, T for temperature and Tau for confinement time. So how long we can hold the energy inside the plasma in the tokamak, because if you put the energy in and it all falls out again straight away, it's not super helpful. So you have to increase the confinement time to get that going, make ignition happen and keep your plasma burning.
James - You following us so far? Still with us? Well, don't worry if the answer is no because after her explanation, Hannah suggested I consolidate my knowledge by roleplaying as a tokamak engineer on earth in the far future. Keen to take up the challenge and prove what I'd learned, I tried my hand at the mobile game which thrusts the player into the hot seat of a futuristic power plant...
James - <Sci-fi music fades in> 'The energy shortage of the 21st century has been overcome. The solution: fusion energy. Fuel is heated to its plasma state at 200 million degrees at which it fuses and releases vast amounts of energy. Powerful magnets hold the plasma inside the power plant. You are the operator of a typical fusion power plant in 2103. As operator, you must drive the machine to the ideal fusion conditions. You control strong magnets to cage the plasma in the steel vessel, a powerful microwave heating system enabling the plasma particles to fuse, and a microwave cannon to blast magnetic islands. You've got to look after the temperature gauge, which goes up to 200 million degrees, and the confinement gauge where you adjust the magnetic power to keep the confinement at the right level.'
James - Right. Okay, I think I'm ready to give this a go. So I want to raise the magnet power. Oh, the confinement is way too high. Sorry. Okay, we'll increase the temperature, but that in turn brings the confinement quite low. Up comes the magnet. Okay, well done, core temperature is rising again. Phew. So the trick is to raise the magnet power and the heating power sort of evenly. We're up to 70 million degrees in my fusion reactor now things are going pretty well. Got to zap those magnetic islands with my microwave cannon. We're going to whack the heating power onto full now and raise the magnet power in turn. Ticking over 190 million degrees, 197, 200. 'Well done. You succeeded in driving the machine to the ideal fusion conditions.' Well,
James - Pretty pleased with myself there. Now before I let Hannah go, I wanted to ask her about Ian's query regarding how these obscene temperatures can be maintained while at the same time being able to extract energy from the fusion reaction.
Hannah - We come to another of the technological challenges, which is capturing the energy that comes out. So you want to make sure that, in the walls of your tokamak, you have the right kind of elements that can capture these neutrons, slow them down, extract the energy, and then that also feeds back into tritium breeding because you can use those neutrons then to react with the lithium to produce tritium. So it kind of makes a nice, neat puzzle. But I mean these are still big technological challenges. The engineering of how that needs to be done is a work in progress.
James - To be able to withstand these extreme conditions, what materials are tokamaks made of?
Hannah - So another technical challenge: material science. A big point of research and not just at tokamak energy but organisations globally looking at different options for how to create these plasma facing components, like the first wall inside the tokamak. So there are different options. Heavy metals with very high melting points, things like molybdenum, tungsten, they can withstand the highest heat loads if a plasma touches the wall. But the problem with them is that if they do get into the core of the plasma, because they're so big, they can end up radiating away a lot of the energy which, if we want to keep the confinement time up, is not great again. Other options are lighter elements right at the other end of the periodic table; boron, lithium we've already mentioned. But because they're light, if they get inside the plasma, they don't do as much damage as it were to the heating, to the maintaining of that temperature.
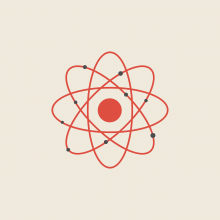
23:38 - Fusion energy research rewards science
Fusion energy research rewards science
Brian Appelbe, Imperial College London
Still with us, Imperial College's Brian Appelbe...
Chris - As I listened to what Hannah said, Brian, and what you told us at the top of the programme, I'm increasingly coming to the view that this is less a physics problem now and more a material science and an engineering challenge. Is that fair or are there still quite a lot of scientific issues to solve?
Brian - What I would say is because we've had successes on the scientific side over the last few years, such as what happened at the National Ignition Facility in America, we are beginning to think more about the engineering challenges. So we still have lots of physics that we need to do to make sure we fully understand how these plasmas behave. But there is a confidence building that we need to start thinking about the next set of problems about how we recover the energy, how we drive these reactions. I think we can straddle both of these challenges.
Chris - Have we created those sorts of conditions on Earth before or are we into uncharted territory trying to make things at 200 million degrees?
Brian - So I think for me personally and for a lot of my colleagues that's the really exciting thing. That when we step back, at the end of the day, what we're doing is we're creating these unique plasmas on earth. It's the hottest substance that we can create and therefore we're able to study it in the laboratory. And what that can do is it can tell us a lot of things about, say, how the universe behaves, how things like supernovae evolve, and how elements are formed in the universe. So whilst trying to achieve the commercial goals of fusion energy, we are still doing fundamental science.
Chris - How will scientists be trying to tackle this? Is this all about computer modelling? Is this just building really powerful computer systems that can work out, when we put things under this enormous strain and pressure and we scale it up to the sorts of sizes that they're talking about, then trying to work out where the particles are trying to go and how fast, and therefore how much energy they're dissipating. Is it basically a computer modelling problem?
Brian - Computers are very, very helpful and we use the world's most powerful computers for doing simulations of these plasmas. But the fundamental issue really is that when you are trying to do fusion, you always are trying to balance a pencil on its point. We have all these instabilities that we have to fight to keep our hot plasma confined. And so the computer simulations help us to understand how we can keep the pencil balancing on its point. But at the end of the day, computer simulations are not infallible. We have to make sure that they're correctly predicting the outcomes of the existing experiments that we can do.
Chris - Do you think George Freeman's going to get his dream in 2040? There'll be a plant running, it will be a net producer of energy?
Brian - If he is willing to support his dream with whatever cash is required, then yes. I always think of the moon landings in 1960. JFK said at the start of the 1960s, America will put a man on the moon by the end of the decade. And he didn't talk about the commercial aspects of it. That was the goal. Get a man on the moon. And I think that's what we should be doing with fusion.
Chris - He also said, "we don't do it because it's easy, we do it because it's hard." And this is a sort of similar problem, isn't it?
Brian - I think so. Yes. I feel we need that attitude.
Chris - And presumably in the course of solving this very hard to solve problem, a bit like the Reimann hypothesis has made mathematicians develop all kinds of exciting new maths to find prime numbers, and they still haven't won the prize. They nevertheless have done some exciting maths doing it. Are we discovering some exciting physics along the way?
Brian - We certainly are. We're discovering lots about astrophysics, also technology developments, things like laser developments, high temperature superconducting magnets. There's lots of technologies that are required for fusion whereby it's the investment from fusion that's driving the progress.
Comments
Add a comment